The Future of Neuroscience is Worms
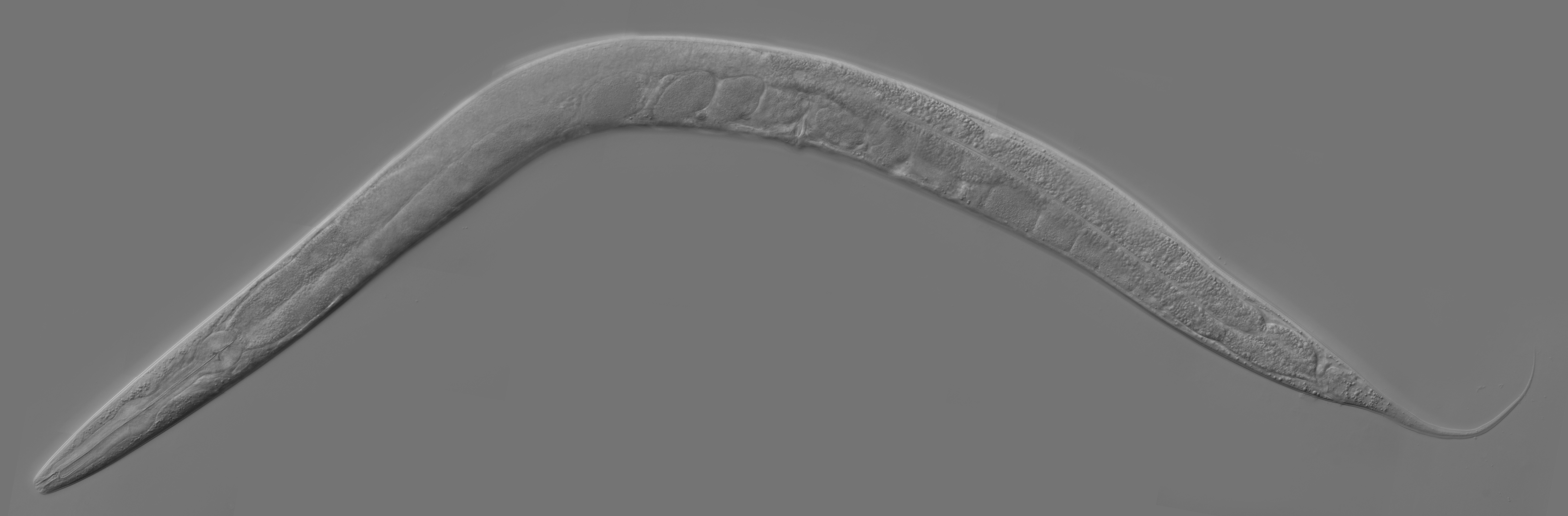
Neuroscience is a field that I believe just about anyone can get fascinated by. We are all interested in how we came to be and because we all have brains we are naturally interested in understanding the subjective, wonderful experiences that come with them. And because there is this built-in repository of firsthand experience that we all have with our own brains, I think neuroscientists have a leg up on other science communicators because their audience will have something personal to relate to, something that cannot be taken for granted when discussing supernovas, parasitic fungi, or enzyme kinetics (although those things can be awesome in their own right). I am also interested in brains and, like many other scientists whose focus is not neurosciecne, I am a bit of an armchair neuroscientist and take great pleasure in listening to the latest neuroscientific findings. [ For a great background I recommend Charlie Rose' Brain Series, as well as Eric Kandel’s In Search of Memory and V.S. Ramachandran’s Phantoms in the Brain. ] A few days ago I was able to enjoy a top quality lecture from Professor Cori Bargmann who is using model organisms to understand how genes, via neurons, control behavior.
I’d seen Professor Bargmann talk a few years back when she came to Mount Sinai and delivered a lecture that I would rank as one of my all time favorites. That lecture had revolved around using the model organism C.Elegans to figure out how an organism was able to detect and respond to environmental stimulus and she had presented data identifying the genes involved in sensing and responding to the concentration of environmental oxygen. She identified the neurons involved in sensing oxygen by looking where along an oxygen gradient the worms would prefer to migrate.The beauty of model organisms is in being able to have a completely “vertically-integrated” story where a large behavior question affecting an entire organism can be traced from the top (behavior) all the way through the tissues, the specific cells, and eventually to the specific genes and molecules that are implicated in the control of that behavior. While many (most? - the vast majority? all?) traits are multigenic, the beauty of the model organism is the exquisite spatial and temporal control that can be achieved to isolate out the specific contributions of specific genes at specific times in specific tissues. This fine-level detail was exactly what Sydney Brenner set out to achieve when choosing C.Elegans as a model system and his choice has paid off handsomely time and time again. When someone gives such a stellar talk once, the expectations are quite high, but the second time around turned out even better.
The subject of Professor Bargmann’s talk was generally the same: the use of a model organism to understand the neurobiology of behavior, but she set the stakes even higher than before. She said, to paraphrase, “I am interested in the human brain. I am interested in understanding the behavior of my husband and of my graduate students. I want to use the worm as a means to unlock the understanding not only of the worms brain, but also of the human brain”. This is a big claim. While we can imagine basic aspects of physiology are conserved among species, can there really be such conservation of behavior circuitry? Really? In a worm? With only 300 odd neurons? I found myself skeptical of the claim despite being aware of the strong precedent of using model organism to understand physiological processes of collections of neurons (including a fellow Rockefeller faculty member). Can a worm help us understand our spouses or our students? Hmmm.
Although she focused on three stories, she spent more time on one particular set of experiments. The Bargmann lab had set up a series of microscopes that allows investigators to track worm activity over long periods of time. Worms have two characteristic behaviors of motion: periods of lounging around where they move but remain in the same area and periods of running around where the worms forage rapidly all over the plate. Videos cameras can be automated to track the worms and categorize their behavior using their rate of motion and the curvature of their path of motion. The ratio and periodicity (switching-between) the two types of behaviors is statistically set: Wild Type worms spend the same amount of total time in the two states and also switch between them at the same frequency. The lab then systematically went about looking for genes involved in regulating these behaviors by knocking out all of the known neural receptors one by one and screening each of the mutants for changes in behavior.
The genetic screen identified a set of candidates that affected the ratio of lounging to running, and they chose the two genes that had the most extreme affects - one biasing toward lounging, the other biasing towards running. What happens next is absolutely remarkable and highlights the power of the model system; it also highlights a few features of neural connectivity that I was unaware of. First, the group was able to identify the exact cells in which these receptors were located. The exact cells. In the course of discussing how this was done, Bargmann mentioned the distributions of the two types of receptors (they were both receptors), and they were in the range of 5-10% of all neurons (15-30 neruons out of ~300). I found this interesting since as a non neuroscientist i would have naively assumed that the receptor profile of all of the cells would be the same and only the connectivity between cells dictates activity. Not so: lineage-specific expression of genes is very important. Upon reflection I should have remembered that this neuron-specific expression is very important for odorant receptor migration and, I believe, neural stem cell development/migration. In any event, armed with the knowledge of how the genes affect behavior and where they are distributed they systematically knocked down the genes in each of those individual cells and found the exact cell responsible for the phenotype. Wow again. Not content with this, they set out to experimentally replicate the exact way in which the interaction occurred. One of the genes was involved in the recognition of a neurotransmitter and they were able to modify the receptor cell with a light-activateable version of the receptor that could artificially stimulate the specific cell upon the application of red light (optogenetics) and in doing so were able to modulate the relative lounging vs. running behaviors. All of this data was needed to verify that the neurons were interacting with one another in the exact way that had been proposed.
In the end what was achieved? The data I have been describing was used to construct a control network of the worm’s behavior where every neuron in the brain responsible for a behavior was mapped. The control structure for the entire behavior could be reconstructed as a set of interactions between neurons and even the specific molecular interactions governing those behaviors. Remarkable. And because the receptors and neurotransmitters involved in the C.Elegans brain are conserved in human brains as well, it is likely that the control structures governing neuronal interactions (and therefore behavior) understandable in C. Elegnas brains will have direct implications for the control structures in human brains as well.
When I walked into the lecture I would have been very skeptical of the utility of C.Elegans for directly tackling problems of human brain behavior and physiology. I would have thought that a better way would be in the fast-growing world of brain imaging and the possibility of the application of optogenetic techniques in “higher” model organisms. After seeing this talk, however, I am amazed again at the C.Elegans community and of Professor Bargmann in particular, in being able to apply genetic tools to understand aspects of the brain that would be prohibitively complicated in almost any other imaginable setting. Bravo.